The ‘quantum’ hype and public funding in Switzerland
In recent years and months, 'quantum' has become the new buzzword. In the area of research and innovation, there is almost no way of getting around it. But what are the fundamental ideas associated with this term? And how well positioned is Switzerland in quantum science and quantum technologies?
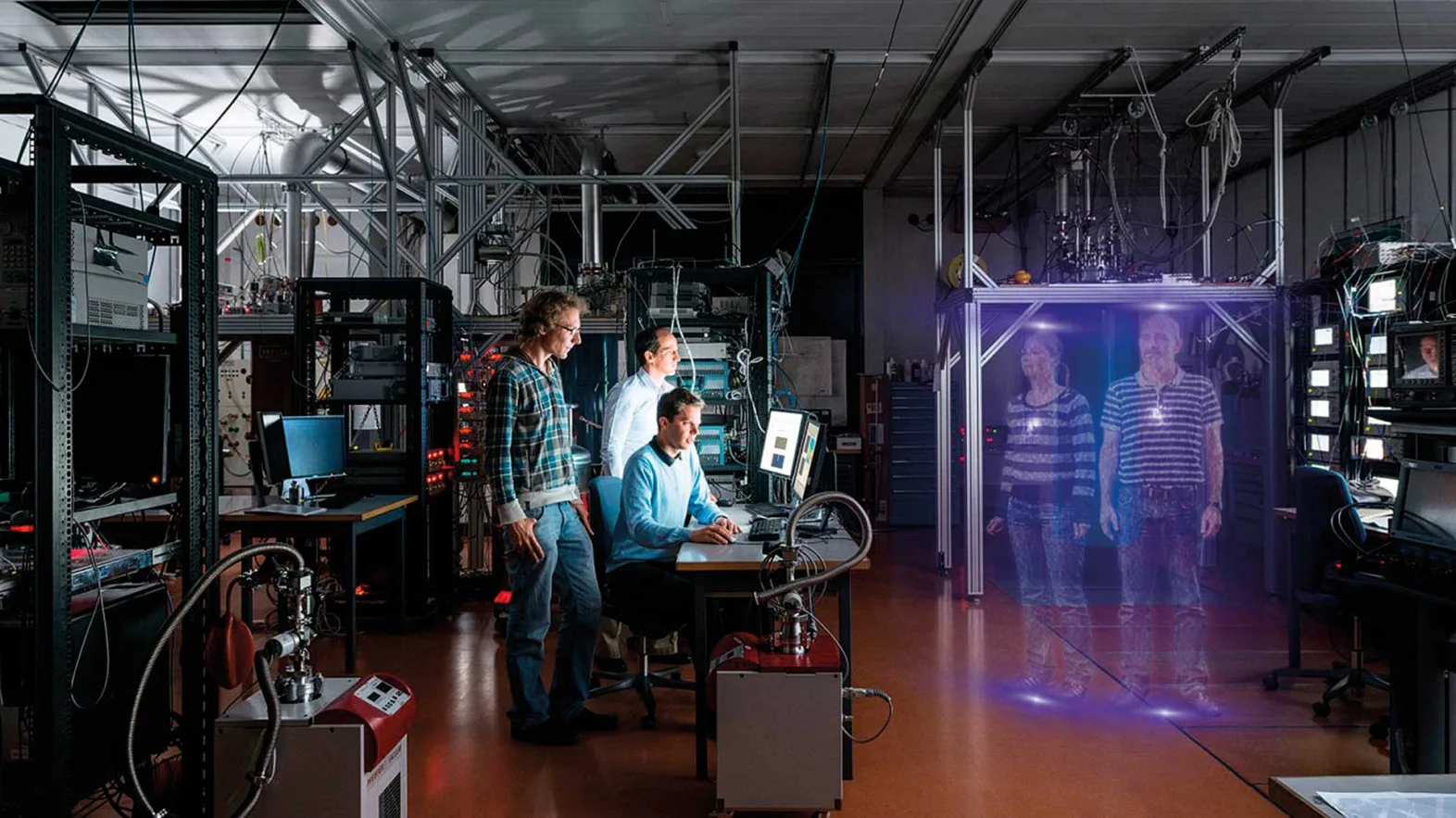
In our ordinary lives, the rules of 'classical' physics can be used effectively to describe the behaviour of nature. For example, we reasonably know what to expect if we send current of a certain intensity through an electromagnet or if we shine light through a lens with a certain focal length.
Quantum mechanics, nature expressed on a very small scale
When researchers began to study the behaviour of nature on a very small scale in the early 20th century, they soon discovered that atoms and light do not obey known rules. Their observations literally defied common sense. It took about 25 years to develop a set of rules for quantum mechanics that could be used to describe nature on a very small scale. However, no one really fully understood what they were observing. And we still don’t.
Very small,...
Quantum mechanics comes into play as soon as we get down to roughly the size of atoms. Atoms consist of a positively charged nucleus and a negatively charged electron shell and have a radius of one ten-millionth of a millimetre. The atomic nucleus is another 20,000 to 150,000 times smaller than that. If we could inflate an atom to a billion times its size, the pinhead-sized atomic nucleus would be orbited by similarly sized electrons at a distance of about 100 m, with literally nothing in between. But this picture is in some ways inaccurate: an atom and its building blocks are not objects in the traditional sense, but rather quantum phenomena - in other words, they are quite different. Quantum objects are very small and/or very cold. Examples include molecules, atoms or elementary particles.
...in packets,...
It is possible to heat a pot of water evenly and it is possible to apply the brakes on a bicycle so that it slows down steadily. In the quantum realm, however, energy cannot be continuously added to or removed from quantum objects in the manner illustrated by these examples from classical physics. It can only be done gradually: Energy is only absorbed or released by quantum objects in suitable packets, which are referred to as quanta (Figure 1).
Thus, such energy packets often manifest themselves as a photon ('light particle') (Figure 2). Each atom has characteristic light frequencies or wavelengths that it is able to either absorb or emit.
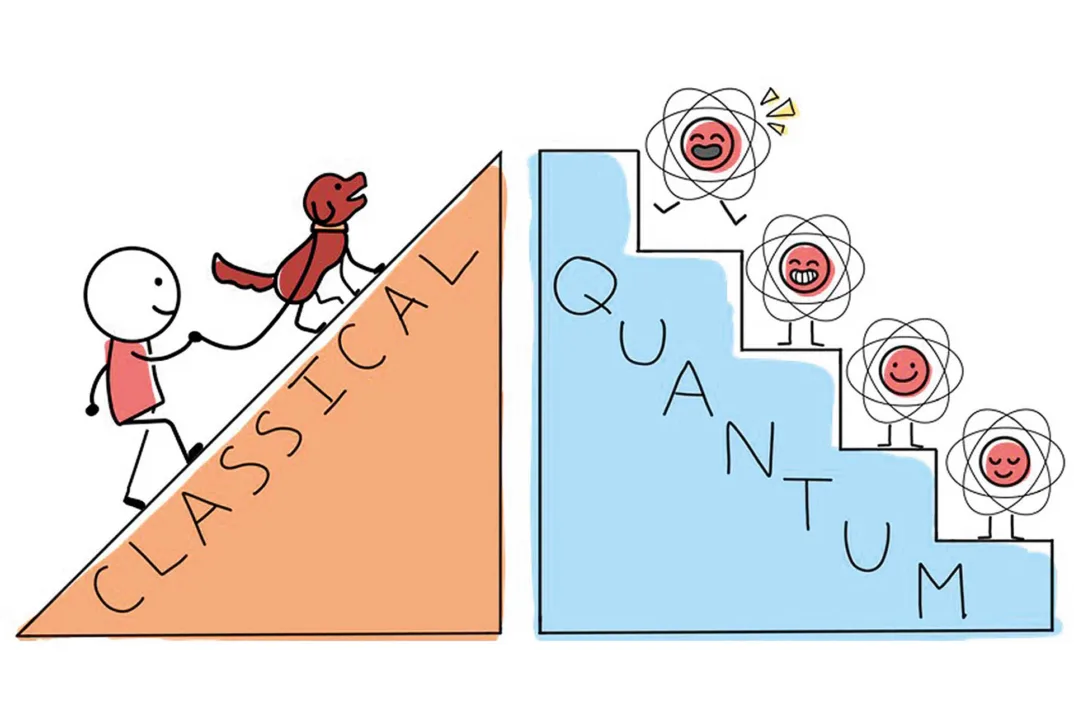
...capricious, ...
In classical physics, if you know the rules that govern a process, you can calculate exactly what state a given system will be in at a given point in time. Thanks to Newton's laws, for example, we know very precisely where the planets will be in the future and how fast they will be moving then. For quantum objects, on the other hand, the superposition principle applies: in other words, quantum objects are in all states and locations possible for them simultaneously. Only when they are measured do they determine where and in which state they are observed. All we can do is predict a probability that a certain value will be observed.
In addition, quantum objects are subject to the uncertainty principle. This means that it is not possible to precisely measure both variables in a given pair for the quantum object being observed. All we can do is reduce the level of inaccuracy down to a certain threshold. The more precisely the value of one variable is known, the less precise our measurement of the other variable will be. Examples of such variable pairs are location/momentum, energy/time, spin/orientation, etc.
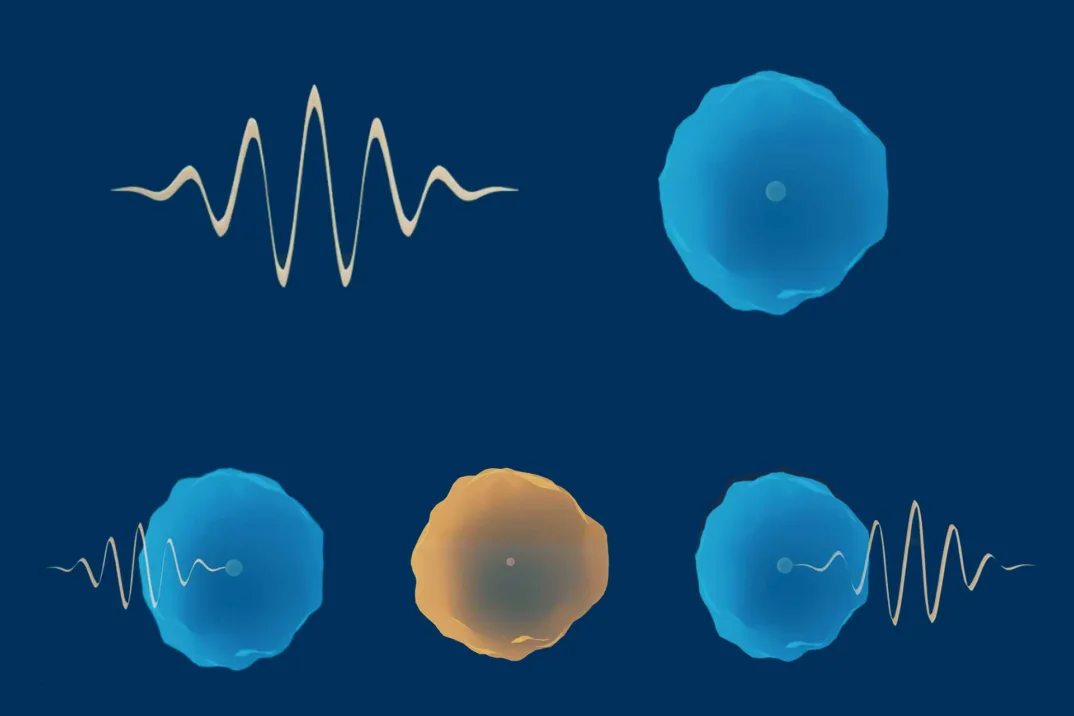
...bizarre.
Quantum objects have properties that contradict our experience:
- Superposition: Quantum objects simultaneously exist in all states that are possible for them. They only settle on one when they are measured.
- Probabilistic: No exact predictions can be made about quantum objects, only probabilities of observation can be given.
- Uncertainty: Measured pairs of variables cannot be determined with precision.
- Duality: Depending on how one performs the measurement, quantum objects show up as waves or as particles. In a certain sense they are both.
- Entanglement: If two or more quantum objects interact with each other, their properties are linked (entangled) in such a way that it is no longer possible to describe the whole system on the basis of individual quantum objects alone. If entangled quantum objects are separated spatially, the system retains its overall properties. If one quantum object is manipulated, the other will react instantaneously, even over arbitrary distances, in order to preserve the overall properties of the system. (Figure 3).
- Tunnelling: Quantum objects can overcome ('tunnel through') obstacles that would be too 'high' for them according to the rules of classical physics.
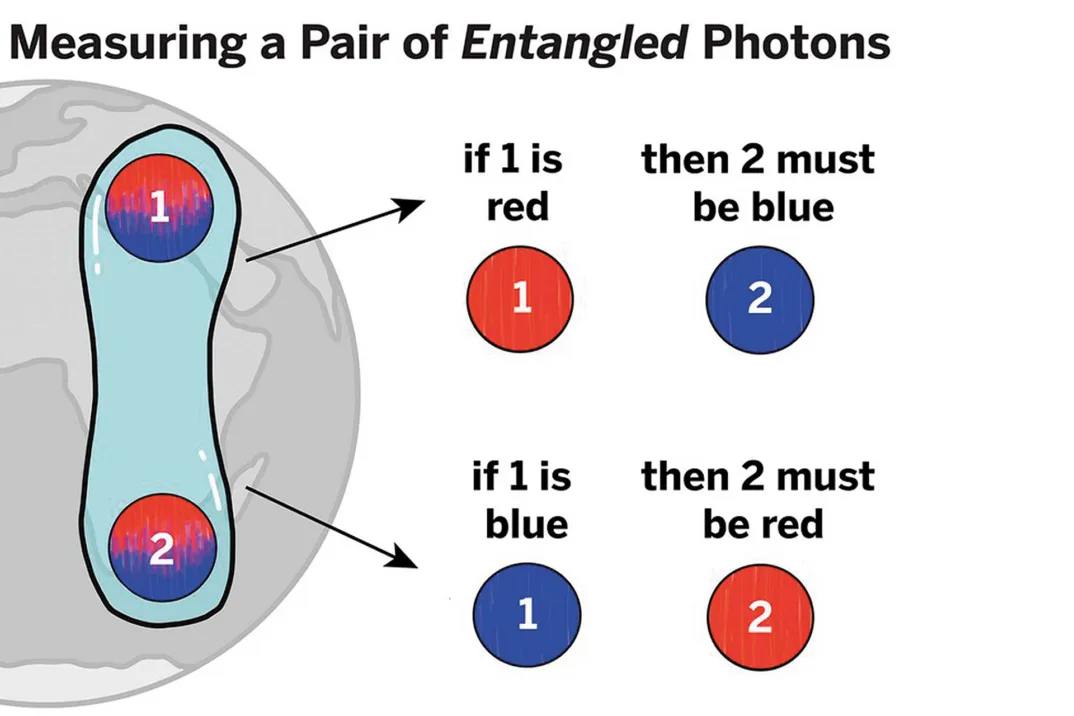
‘Old’ quantum technology
Quantum technology can be found everywhere in our daily lives. Be it the semiconductor transistors used as building blocks for computers, or lasers and magnetic resonance imaging: all these (and other) techniques rely on our ability to collectively manipulate quantum objects.
‘New’ quantum technology
Technologies that manipulate individual quantum objects are colloquially referred to as the 'second revolution in quantum technology' or 'Quantum 2.0'. Strategically important fields include the following:
- Quantum sensors and measurements: The quantum realm is opened up by sensors that measure individual quantum phenomena.
- Quantum computing: Today's conventional computers are based on a digital (binary) logic for data storage and computation. From a technical standpoint, this is achieved by switching the positions of suitably connected transistors 'on' and 'off'. However, if we use special quantum objects (referred to as Qbits) instead, our logic changes from binary to the superposition principle, where all of possible quantum states that the object can take overlap. In theory, this will constitute a ‘quantum leap’ in computer performance over the most powerful computers currently available.
- Quantum communication: Here, the entanglement of delocalised quantum objects (mostly photons) is used to transmit data keys. This technology is already available. In principle, this type of communication cannot be intercepted, as any 'eavesdropping' by third parties influences the signal.
Because of the various properties of quantum objects described above, achieving these outcomes are easier said than done and are the subject of current research. For example, estimates of when the first usable universal quantum computer will be available vary between five and 30 years.
Quantum technology: why the hype?
'Quantum 2.0' means technological progress that will presumably bring about the same kind of radical upheavals as industrialisation did after the steam engine was invented.
For today's highly digitalised global society, the market for the transition from 'traditional digital' to 'quantum' is huge and the economic and political stakes are high. On the one hand, quantum communication and cryptography will allow data to be securely stored and transmitted. On the other hand, in addition to boosting R&D potential (e.g. in pharmaceuticals, medicine, development of materials, logistics, manufacturing technology), quantum computers will be able to bypass and crack data encryption and security protocols that were developed using traditional computer technology.
This is also the reason why many countries are currently engaged in very fierce competition for 'Quantum 2.0': Whoever can achieve breakthroughs first will have a major edge in terms of economic and security policy. The companies involved anticipate billions in sales and profits. This is why many countries are allocating considerable public funds to this area of research: China leads the way in terms of funding (USD 15 billion), followed by the EU (USD 7.2 billion). Most EU funding comes from Germany (USD 3.1 billion), France (USD 2.2 billion), the European Commission (USD 1.1 billion) and the Netherlands (USD 0.9 billion). (Source: World Economic Forum, State of Quantum Computing: Building a Quantum Economy, 13 September 2022).
The multilateral initiative 'Pursuing Quantum Information together' operates in this context. Twelve countries (currently US, AUS, CA, DK, FI, FR, DE, JP, NL, SE, CH, UK), all sharing common values such as freedom, democracy and transparency, are engaged in technical discussions to develop a common framework for international cooperation in quantum technology research. The aim is to optimise research while at the same time safeguarding the economic and security interests of all participants. A Swiss delegation of specialists from SERI takes part in these discussions.
And what about Switzerland?
How well is Switzerland positioned in terms of quantum science and technology? While China, the USA, Russia and Germany are the most active countries when it comes to quantum publications, Switzerland is ahead of Germany, the UK, Austria and the USA in terms of impact. This is also due to the fact that Switzerland has been investing in quantum research for over two decades.
Since 2001, the federal government has been funding quantum research through National Centres of Competence in Research (NCCR), which have received roughly CHF 50 million each over the past ten to twelve years:
- NCCR Nanoscale Science, 2001–2013, Leading House: University of Basel,
- NCCR Quantum photonics, 2001–2013, Leading House: EPFL,
- NCCR QSIT, 2011–2022, Leading House: ETHZ, University of Basel,
- NCCR SPIN, 2021–2031, Leading House: University of Basel
Swiss Quantum Initiative
This bottom-up initiative was launched by SERI and is being implemented by the Swiss Academy of Sciences (SCNAT). It is expected to have an impact from 2023 onwards. Thanks mainly to federal and university funding provided through the NCCR, this initiative is intended to consolidate Switzerland's position in quantum technology and improve its international competitiveness by providing additional opportunities for cooperation and funding at national level. The initiative includes: targeted reinforcement of research through competitive calls for proposals; the development of relevant study programmes; partnerships with industry for knowledge and technology transfer; and international cooperation.
Quantum Transitional Call
Under the EU Framework Programme for Research and Innovation, Horizon Europe (HEU, 2021-2027), Switzerland is currently a non-associated third country and is thus excluded from participating in HEU calls relating to quantum technology and other key technologies that the EU deems strategic (space, high-performance computing).
To compensate for this, SERI gave the Swiss National Science Foundation (SNSF) a mandate to implement a national 'Quantum Transitional Call', which was launched in 2022.
Measures in the space sector
In a bid to overcome the barriers resulting from Switzerland’s third-country status in Horizon Europe, the Federal Council adopted a series of transitional and compensatory measures, some of which can be implemented by the European Space Agency (ESA), due to the fact that Switzerland is a founding member. As a result, Swiss actors will continue to take part in space projects relating to quantum science and technology.
Fresh talent needed
While the continuation of quantum research certainly requires funding, this is only the starting point. It is just as important to have creative, enthusiastic researchers and developers who are well connected in the international quantum science community. At the same time, fresh talent is needed - and progress is already being made on this front, as illustrated by the first study programmes in quantum technology. Switzerland's economic prosperity will depend on how well schoolchildren's interest in quantum technology can be awakened and whether specific training programmes can be established to qualify them.
Contact
Author
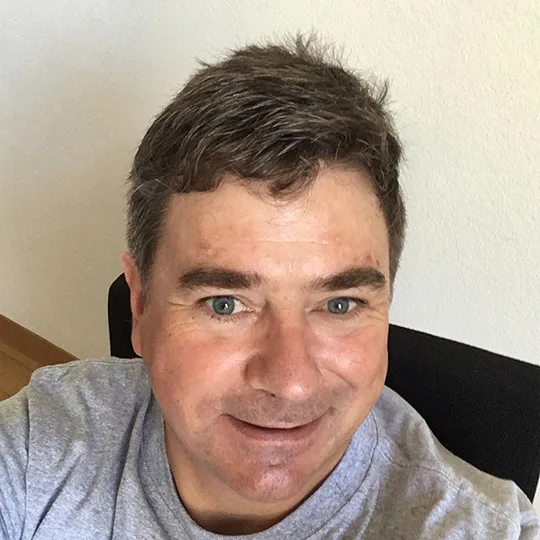